Origins of organics in water and how to reduce
cut lineUltra-pure water is usually produced by the multi-stage treatment of potable mains water. Organic compounds in the feedwater are both naturally occurring and man-made. Naturally occurring compounds are derived from the decomposition of vegetation and are a complex mixture of humic and fulvic acids and tannins. In addition, there are bacteria and their by-products. Man-made compounds include industrial waste from detergents and oils; fertilisers, herbicides and pesticides from agriculture and a wide variety of contaminants from domestic waste.
The concentrations of impurities will vary with the area and season and amounts of rainfall. As the water is rendered potable, many of the contaminants are removed, but some others may be introduced, such as plasticisers from pipework systems or bituminous coatings from tanks. Further compounds may be produced during the water treatment process as a result of the action of chlorine or ozone. These include low molecular weight halogenated species such as chloro-form which are difficult to remove.
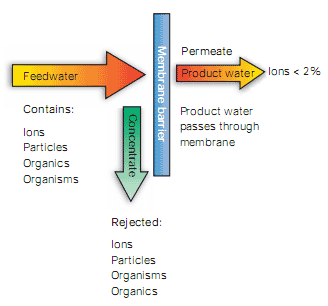
Figure 1 Reverse osmosis (RO) pressure-driven deionization
Organics in the feedwater may be present in solution or as colloids or larger particulates. They may be hydrophilic in nature, such as alcohols, or hydrophobic, such as aromatics. Molecular weights may range from less than 100 daltons up to 4000 daltons or more. Total organic carbon levels in potable water range typically from 0.1 to 4 ppm.
REDUCING TOC LEVELS
The principal technique used to remove the majority of organic compounds from potable water is reverse osmosis (RO). Water is pumped into a cylindrical pressure vessel containing a spiral of semi-permeable membrane. The membrane rejects over 95% of ions and organics with molecular weights greater than 100 daltons. It also provides a barrier to bacteria and particulates. However, RO is less effective at removing low molecular weight organics such as alcohols and halomethanes. The purified water passes through the RO membrane to form the permeate. The contaminants remain in the residual water (the concentrate) which is flushed continuously to drain. The characteristics of purification by reverse osmosis are illustrated in Figure 1 and a module construction is shown in Figure 2.
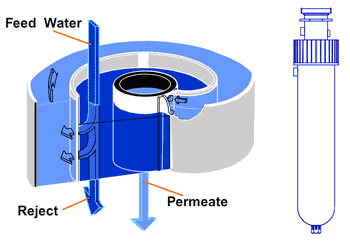
Figure 2 Spiral wound RO membrane
Additional technologies are used to remove trace organics from the water. Ion exchange resins will trap ionised or strongly polar organic compounds, such as acids or salts. Mixed beds of anion and cation resins are effective, however the addition of organic scavenging resins may enhance removal performance. Activated carbon, in the form of granules or briquettes, will adsorb any remaining trace organic compounds from the water. Its effectiveness will depend on the pore size of the carbon and the nature of the organics. The pore size of carbon is selected to maximise the adsorption of low molecular weight compounds and as such serves to complement the RO performance. The effectiveness of activated carbon is restricted by the weak nature of the Van der Waal’s forces holding the organics in the micropores of the granules. The removal of trace organic compounds from water by activated carbon is thus never complete. Additionally, organics may be displaced as the adsorbent becomes saturated or by the presence of more strongly adsorbed species. A disadvantage of all media is that they can also be a source of organic leachates which can re-contaminate the purified water. Great care is therefore needed in the selection and pre-cleaning of resins and other media.
To eliminate the final traces, the organic contaminants are oxidized to a form that can be removed more effectively. In large industrial water purification plants, oxidation by UV radiation or ozone or a combination of the two is used, for example, to provide wafer rinse water for the electronics industry. Of these methods, UV irradiation is by far the most common and is also most suitable for adaptation to the laboratory scale.
Ultraviolet oxidation of organics on a laboratory scale uses special low-pressure mercury discharge lamps. The relative intensities of the spectral lines emitted by this type of discharge are shown in Figure 3. Over 90% of the light is at the germicidal wavelength of 254nm while a small fraction (approximately 2%) is emitted at the 185 nm wavelength. Normally this light is absorbed by the quartz window of the lamp; however, special lamps with high transmissivity quartz sheaths enable this radiation to be utilised.
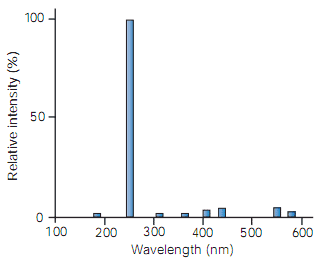
Figure 3 Low-pressure mercury lamp
Ultraviolet light at 185 nm is sufficiently energetic to react with organic compounds in two ways :
- Directly by cleaving organic bonds.
- Indirectly via the generation of hydroxyl radicals.
Bond | Dissociation energy (kcal/mole) | Maximum wavelength for dissociation (nm) | Dissociation possible by 185 nm radiation |
---|---|---|---|
C-C | 82.6 | 346.1 | Yes |
C=C | 145.8 | 196.1 | Yes |
C-F | 116.0 | 346.5 | Yes |
C-CI | 81.0 | 353.0 | Yes |
C-N | 72.8 | 92.7 | Yes |
C=N | 147.0 | 194.5 | Yes |
N-O | 48.0 | 595.6 | Yes |
C=O | 176.0 | 162.4 | No |
Table 1 Dissociation of bonds by UV radiation
As shown in Table 1, radiation at 185 nm is able to break most organic bonds, thus splitting large molecules into smaller fragments. However, the main mechanism is its reaction with dissolved oxygen in the water to produce OH radicals. These are highly oxidising, reacting with organics present to produce organic acids, typically lower molecular weight species such as acetic acid. On further, prolonged exposure to the UV light these acids are oxidised to carbon dioxide. The acids and carbon dioxide can all be removed from the water by anion or mixed-bed ion-exchange resins. Figure 4 shows examples of the effectiveness of UV light at reducing TOC levels. Depending on flow and lamp intensity, TOC is reduced by 60% to 90%. In addition to removing organics present in the feedwater, purification systems must also minimise the leaching of contaminants from internal system components. This is somewhat difficult due to the general requirement to use plastic components to minimise any effect on the inorganic purity of the product water. Careful selection and testing of materials are required. Pure polyethylene, polypropylene and fluorocarbon polymers are the preferred alternatives.
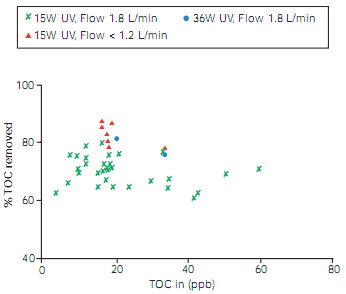
Figure 4 % TOC removed by UV radiation followed by deionisation
Plastics such as nylon with water stable plasticisers (for example benzene sulphonamides) must be avoided. The TOC content of various types of water is illustrated in Figure 5.
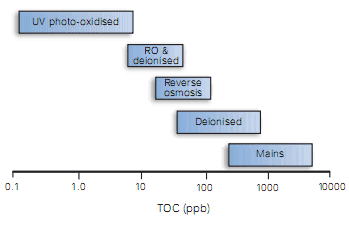
Figure 5 Organic purity of different types of water