Water purification
cut lineMethods of water purification
- Distillation
- Ion exchange
- Reverse osmosis
- Activated-carbon adsorption
- Microporous filtration
- Ultra-filtration
- Photo-oxidation
Distillation
Distillation is a long established process for water purification in which water is heated until it evaporates and the vapor condensed and collected. The equipment is relatively inexpensive but it is very energy intensive - typically it uses 1kW of electricity per liter of water produced. Depending on the design of the still, distilled water can have a resistivity of around 1 MΩ-cm and will be sterile when freshly produced if purpose built equipment is used, but will not remain so without very careful storage. Moreover, volatile impurities such as carbon dioxide, silica, ammonia and a variety of organic compounds will 'carry over' into the distillate.
What are the drawbacks of distillation? Distillation only produces purified water slowly. It is not an on-demand process. Due to this, a quantity of water must be distilled and stored for later use. This storage of the distillate can be problematic if the container in which the water is stored is not made of an inert material. Ions or plasticizers will leach out of the container and re-contaminate the water. In addition, bacteria grow very well in water that has been standing for some time.
To maintain sterility, sterile storage bottles are used and the collected water autoclaved, but once the bottle is opened it is exposed to bacteria and contamination begins. In hard water areas stills require frequent acid cleaning, due to scale build-up, unless the feedwater is pre-treated by softening or reverse osmosis.
Ion exchange
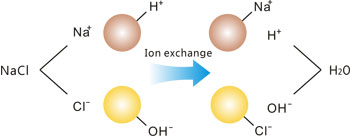
Ion exchange is widely used in laboratories for providing purified water on demand. Laboratory deionizers invariably incorporate mixed-bed cartridges of ion exchange resins that are either returned to a regeneration station for recharging when they become exhausted, or else discarded.
Anions and cations in the feedwater are removed by the ion exchange resins and are replaced by hydrogen and hydroxyl ions from the resin. The hydrogen and hydroxyl ions combine to form water molecules.
How does ion exchange work? Ion exchange functions by exchanging hydrogen ions for cationic contaminants and hydroxyl ions for anionic contaminants in the feedwater. The ion exchange resin beds are made up of tiny spherically shaped beads through which the feedwater passes. After a period of time, cations and anions will have replaced most of the active hydrogen and hydroxyl sites in the resins and the cartridges will need to be replaced or regenerated.
What are the benefits of ion exchange? Ion exchange has many advantages over distillation for the production of purified water. First of all, it is an on-demand process; the water is available when it is needed. Secondly, when using high purity resin materials, effectively all the ionic material will be removed from the water to give a maximum resistivity of 18.2 MΩ-cm (at 25ºC). Tiny fragments of the ion exchange resin materials can be washed out of the cartridge by the water passing through it. Therefore, ion exchange should be used in conjunction with filters if particle-free water is required. As bacteria will grow rapidly in stagnant water, the cartridges may become contaminated if they are not regularly used. The problem is alleviated by frequent recirculation of the water to inhibit bacterial build up and by regular replacement or regeneration of the resins, since regenerant chemicals are powerful disinfectants.
Ion exchange will only remove polar organic compounds from the water and dissolved organics can foul the ion exchange beads, decreasing their capacity. Where organically and inorganically pure water is needed, the combination of reverse osmosis followed by ion exchange is particularly effective.
There have been many attempts to overcome some of the limitations of ion exchange and distillation. In some systems distillation has preceded ion exchange - the cartridges last much longer, but the problem of bacteria remains. In some others, ion exchange has preceded distillation - but then the problems of storage and not having water on demand remain.
Reverse osmosis
Reverse osmosis (RO) is a process that overcomes many of the shortcomings of distillation and ion exchange. To explain reverse osmosis let us first look at osmosis. This is a natural process, which occurs whenever a dilute solution is separated from a concentrated solution by a semi-permeable membrane. Water, driven by a force caused by the concentration difference - the osmotic pressure - passes through the membrane into the concentrated solution. The flow of water continues until the concentrated solution is diluted and back pressure prevents any further flow through the membrane.
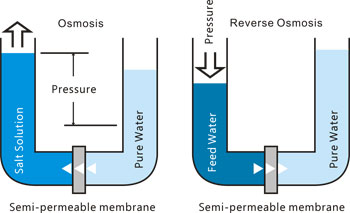
If a pressure greater than the osmotic pressure is applied to the higher concentration side of the membrane, the normal direction of osmotic flow is reversed, pure water passes through the membrane from the concentrated solution and is thus separated from its contaminants. This is the basic principle of reverse osmosis.
In practice, feedwater is pumped into a pressure vessel containing a spiral or set of hollow fibers of semi-permeable membranes. The purified water passes through the membrane to form the 'permeate'. The contaminants accumulate in the residual water, called the 'concentrate', which is bled continuously to drain. The latest generation of polyamide thin film composite reverse osmosis membranes which have replaced early cellulosic membranes, remove 95-99% of inorganic ions, together with virtually all the large non-ionic contaminants and organic molecules with a molecular weight greater than 100. Dissolved gases are not removed.
Thin-film composite membranes are used in all Puring's laboratory reverse osmosis equipment.
Due to this exceptional purifying efficiency, reverse osmosis is a very cost-effective technology for a water purification system. However, it is limited by the relatively slow rate of production and is, therefore, normally used to fill a reservoir prior to use or further purification. Reverse osmosis tends to protect the system from bacteria and pyrogens. It is often combined with ion exchange to considerably improve product water quality.
Adsorption media
Activated carbon, prepared by pyrolizing coconut shells, coal or resin beads removes chlorine by a catalytic mechanism and dissolved organics by adsorption and is often found at two locations in a water purification system. The carbon can be used as granules or more conveniently in block form. As thin-film composite reverse osmosis membranes could be damaged by excessive exposure to free chlorine, and, to a lesser degree, fouled by dissolved organics, activated carbon is often placed prior to the RO membrane to remove these contaminants.
Activated carbon filters are also often placed in the polishing loop of a water purification system to remove trace amounts of dissolved organics, prior to final ion exchange.
Microporous filtration
Microporous filtration membranes provide a physical barrier to the passage of particles and micro-organisms and have absolute ratings down to 0.1 micron; some Puring systems also incorporate "ultra- micro filters" with a rating of 0.05 micron. Most raw waters contain colloids, which have a slight negative charge (measured by the Zeta potential). Filter performance can be enhanced by using micro filters that incorporate a modified surface, which will attract and retain these naturally occurring colloids, which are generally much smaller than the pore sizes in the membrane. Micro-filters with an absolute pore size of 0.2 micron are widely used in water treatment systems. They trap contaminants, including carbon fines from organic adsorption cartridges, resin particles from ion exchange cartridges and bacteria.
The sub-micron filter may be fitted to the dispenser so that the last filter the water passes through before it is used will be the sub-micron filter.
Microporous membranes are generally considered to be indispensable in a water purification system, unless they are replaced by an ultra-filter.
Ultra-filtration
Ultra-filtration uses a membrane very similar in design to reverse osmosis, except that the ultra-filter pores are slightly larger, from 0.001 to 0.02 micron. For pyrogen removal the pores of an ultra-filter should be about 0.002 microns in diameter or less and should exclude all molecules with a molecular weight of 5,000 or larger.
Ultra-filters may be used in a similar way to micro-porous membranes, but may also be set up to allow a small portion of the feedwater to tangentially flush the membrane to minimize the build up of contaminants and the growth of bacteria. Ultra-filtration is an excellent technology for ensuring consistent ultra-pure water quality in respect of particles, bacteria and pyrogens.
Photo-oxidation
Photo-oxidation uses high intensity ultra-violet radiation to destroy bacteria and other micro-organisms and to cleave and ionize any organic compounds for subsequent removal by ion exchange cartridges. Radiation with a wavelength of 254 nm has the greatest bactericidal action, while radiation at shorter wavelengths (185 nm) is most effective for the oxidation of organics.
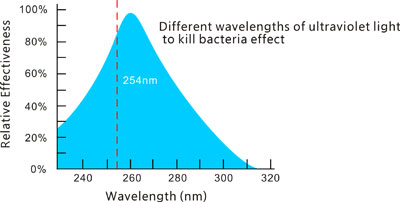